 |
Information box |
The main purpose of this site is to extend the
intraoperative monitoring to include the neurophysiologic
parameters with intraoperative navigation guided with Skyra 3
tesla MRI and other radiologic facilities to merge the
morphologic and histochemical data in concordance with the
functional data.
CNS Clinic
Located in Jordan Amman near Al-Shmaisani hospital, where all
ambulatory activity is going on.
Contact: Tel: +96265677695, +96265677694.
Skyra running
A magnetom Skyra 3 tesla MRI with all clinical applications
started to run in our hospital in 28-October-2013.
Shmaisani hospital
The hospital where the project is located and running diagnostic
and surgical activity. |
|
 |
|
 |
The main purpose of
intraoperative neurophysiologic monitoring is to reduce
postoperative neurological deficits, but more recently it has
become apparent that intraoperative recording of sensory evoked
potentials and electromyographic (EMG) potentials can also aid
the surgeon during many operations. The use of intraoperative
monitoring of sensory evoked potentials and EMG potentials to
reduce permanent postoperative deficits is based on the
assumption that changes in recordable electrical responses occur
as a result of injury, and that the injury is still reversible
at the time of detection if proper surgical intervention occurs.
Monitoring
of brain stem auditory evoked potentials (BAEPs) during
operations in which the vestibulocochlear nerve (cranial nerve
VIII) may be manipulated is now widespread, and the use of
monitoring to reduce the incidence of hearing loss due to
surgical manipulation of the vestibulocochlear nerve is steadily
increasing. However, intraoperative monitoring of visual evoked
potentials (VEPS), has not gained similar acceptance, mainly
because of the technical problems involved in generating a
suitable stimulus. Intraoperative recording of somatosensory
evoked potentials (SSEPs) is valuable during aneurysm surgery
and during other vascular operations, as well as during the
removal of tumors in which the tumor itself or the surgical
manipulation used to remove the tumor can be expected to affect
brain structures that are involved in the somatosensory system
and thus affect the generation of SSEPs.
The most
frequent use of intraoperative monitoring of SSEPs is in
operations involving the spinal cord, in which such monitoring
is now well established. More recently, intraoperative
transcranial stimulation of the motor cortex, by using either a
high-voltage electrical stimulation or a strong magnetic field
impulse in connection with recording of EMG potentials from the
motor system, has been introduced to reduce intraoperative
injuries to the spinal cord.
The
recording of EMG responses from muscles innervated by different
cranial nerves has been shown to be valuable in identifying
cranial motor nerves during operations to remove tumors when the
anatomy has been altered by the tumor or by previous operations;
this is particularly true for operations to remove acoustic
tumors and it has more recently been shown to be of value also
in operations to remove large tumors of the skull base. It has
been possible in a few types of operations to use
electrophysiologic methods to guide the surgeon in the operation
and to ensure before the operation has ended that the
therapeutic goal of the operation has been achieved.
The
objective of intraoperative monitoring of evoked potentials
(BAEPs, SSEPs. and VEPs) when used for the purpose of reducing
permanent postoperative neurological deficits is to detect
changes that occur during the operation. This differs from the
goal of the use of evoked potentials for diagnostic purposes, in
which a deviation from a normal value is of interest. In
intraoperative monitoring, normal values are of little interest;
instead, it is important to obtain a baseline recording from an
individual patient and then to compare the potentials that are
recorded during the operation to that baseline recording. Such a
baseline can usually be obtained after the patient has been
anesthetized but before the operation has begun.
|
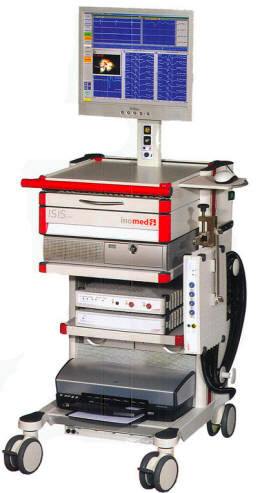 |
 |
Preservation
of the Facial Nerve During Operations in the
Cerebellopontine Angle |
 |
Monitoring of contractions of the facial muscles is
performed during operations in the ccrebellopontine
angle to help the surgeon locate the facial nerve
(CN
VII) when it is
not identifiable visually. Possibly just as important,
this technique makes it possible to determine which
portions of an acoustic tumor do not contain any part of
the facial nerve. This thus allows the surgeon to remove
portions of a tumor without risk of injuring the facial
nerve. Currently most such monitoring involves the
surgeon using a handheld stimulating electrode, which
carries short pulses of electrical current, to probe the
surgical field to identify the facial nerve. Various
methods are used to record the subsequent contractions
of the facial musculature. The facial muscle
contractions that are elicited by irritation and
manipulation of the facial nerve are just as important
as the contractions elicited by electrical stimulation
of the facial nerve for the purpose of assessing
injuries to the facial nerve.
Earlier, it
was customary to have an assistant observe any movement of the
face and then to communicate that fact to the surgeon. More
recently, the recording of EMG potentials from the facial
musculature and the recording of movements of the
face using electronic sensors have also been used to verity that the facial
nerve has been stimulated. The use of EMG recordings makes it
possible to assess the degree of facial muscle contraction
quantitatively, which was not possible when facial muscle
activity was assessed by visual observation of movements of the
face. The capability of making the recorded EMG potentials
audible allows the surgeon to hear when the facial nerve has
been stimulated and thus the surgeon has no need to rely on
communication with an assistant. For this purpose,
only a conventional audio amplifier and loudspeakers need to be
connected to the output of the EMG amplifier. Some commercial
equipment makes use of amplitude-sensing devices that elicit a
tone signal when the EMG potentials reach a preset amplitude.
However, making the (original) EMG signal audible is
advantageous because it provides valuable information that is
lost when such amplitudesensing devices are used. Although the
audible EMG signal provides most of the information that is
needed when monitoring the facial nerve, an oscillographic
display of the recorded EMG potentials is also advantageous, in
that it allows assessment of the amplitudes and latencies of
the recorded EMG potentials. When the movements of the face are
recorded by electronic sensors, the movements can be made to
elicit a sound (horn), but an oscillographic display of the
electrical signals produced by movement detectors has limited
value.
Because the
facial nerve is often split into several fascicles when a large
acoustic tumor has displaced it, it is important that recordings
be made from the entire face. If only portions of the facial
musculature (e.g., the lower face or the upper face) are
monitored, failure to locate all parts of the facial nerve could
result in inadvertent removal of or injury to portions of the
facial nerve: this would result in postoperative paralysis of
part of the face. Usually the record EMG activity is performed between
two
electrodes, one placed
on the forehead and one on the lower face (Fig.-1A), when
monitoring facial function intraoperatively. In addition to
recording activity from all muscles on the side of the face on
which the electrodes have been placed, this particular
arrangement of recording electrodes will also record
contractions of the masseter and temporal muscles. These muscles
are innervated by the motor portion of the trigeminal nerve (portio
minor), and there is the possible risk of mistaking the fifth
(motor) nerve for the seventh nerve when probing the cerebellopontine angle for the facial nerve in operations to
remove large acoustic tumors that have progressed rostral to the
trigeminal nerve. The EMG response to stimulation of the
trigeminal nerve, recorded in the way illustrated in Fig-1A,
however, can easily be distinguished from the response to
stimulation of the facial nerve, because the latency of the
recorded EMG signal differs in the two situations [1.5 ms and 5
to 6 ms. respectively,(Fig-1B). An alternative way
to distinguish between the
response to facial nerve stimulation and that to stimulation of
the motor portion of the trigeminal nerve
(CN
V) is to record from the
masseter muscle using a pair of electrodes connected to a
separate differential EMG amplifier (Fig-1A). This
recording will almost exclusively yield the response of the
masseter muscle, and thus is a good indicator of stimulation of
the motor portion of the trigeminal nerve. |
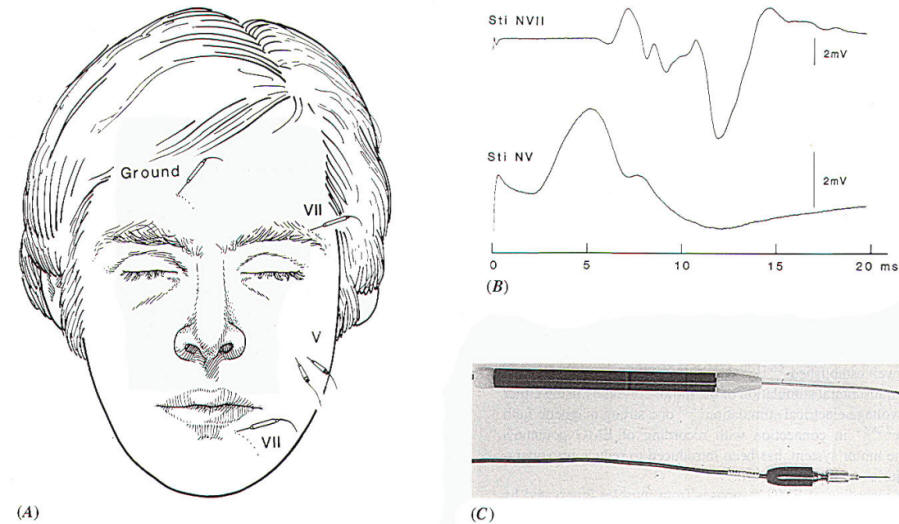 |
Fig-1: A-Electrode
placement for recording EMG potentials
from facial muscles and a separate recording of the
response from the masseter muscle. B- EMG potentials
recorded from electrodes, placed as shown in A,
elicited by electrical stimulation of the trigeminal
and facial nerves in the CPA. C- Hand held
stimulating electrodes for intracranial localization
of the cranial nerves. |
There are
several advantages to using EMG recordings as a measure of
muscle contraction rather than using a single sensor that
records movements of the face. First, EMG potentials from
practically all of the facial muscles can be recorded on a
single channel [Fig-1A),
whereas several sensors are needed to cover the
entire face when movements are being recorded. Second.
recording EMG potentials makes it possible to measure the
latencies of the responses accurately, which enables one to
differentiate between activation of the trigeminal nerve and
activation of the facial nerve (Fig-1B). Third, the amplitude of
the EMG response is roughly a measure of how many
nerve fibers are functioning. and it therefore provides
valuable quantitative information about the degree of injury to
the facial nerve.
There are
essentially two ways that electrical stimulation can be applied
to the facial nerve: one is by using a bipolar stimulating
electrode, and the other is by using a monopolar electrode.
the difference being that the bipolar electrode has a higher
degree of spatial specificity. However, because it is only the
negative phase of the stimulus that is effective, only one of
the two prongs of a bipolar stimulus electrode will stimulate
the facial nerve effectively. Therefore, the orientation of a
bipolar electrode is important. A monopolar. hand-held,
stimulating electrode does not have these disadvantages and
although it is less selective than a bipolar stimulating
electrode it is preferable for intraoperative use (Fig-1C).
A monopolar stimulating electrode is easy to use and its
stimulating power is not affected by its orientation, as is the
case for a bipolar stimulating electrode. By using a monopolar,
handheld, stimulating electrode and having EMG potentials made
audible, the surgeon can probe a large area of a tumor quickly
and "map" the tumor to locate portions of the tumor where no
part of the facial nerve is present so that it can be removed
safely. The use of this technique can often reduce
considerably the time required to remove the tumor because
large portions of the tumor can be removed without risk of
causing injury to the facial nerve. Later during removal of
the tumor, when it becomes important to identify the facial
nerve accurately so that injury to the nerve can be avoided, the
same monopolar stimulator can be used and the area of tissue
that it stimulates can be varied by varying the voltage that is
applied through the electrode.
The
electrical stimulation should consist of negative (rectangular)
impulses of short duration (0.1 to 0.2 ms), and the stimulus
strength should be no greater than necessary to produce a
contraction. Some older types of stimulators make use of large
current and some even make use of direct current. Such
stimulators should not be used because of poor specificity and,
particularly, because of the risk of injuring the nervous tissue
with the electrical current used to stimulate the facial nerve.
Because
shunting of electrical current from the stimulating probe can
vary widely when the surgical field is wet compared to when it
is relatively dry, it is advantageous to use a relatively
constant-voltage stimulator rather than the more conventional
constant-current type of stimulator.
Constant-current stimulation in connection with the use of a
"flush tip" stimulating electrode (i.e., an electrode that is
insulated all the way to its tip) has been advocated by some
investigators. When constant-voltage stimulation is used, the
same amount of stimulus current will flow through a specific
tissue (e.g., the facial nerve), regardless of how much shunting
occurs. If constant-current stimulation is used, the same total
current is delivered, but the amount of current that passes
through a specific volume of tissue depends greatly on how much
current is shunted away; in this case the stimulus strength
depends heavily on whether the field is wet or dry.
When a
facial nerve stimulator is used to identify regions of a tumor
where no part of the facial nerve is present, the stimulus
strength should be set so that it will activate the facial nerve
if the nerve is within a small distance of the tip of a
monopolar stimulating electrodes.
Audible
monitoring (by means of a loudspeaker) of the EMG activity of
the facial muscles that is evoked by mechanical stimulation of
the facial nerve provides valuable feedback to the surgeon
during the delicate resection of portions of an acoustic tumor
that involve the facial nerve. Such continuous
monitoring of facial EMG activity (without electrical
stimulation) is of great value, particularly when the surgeon is
removing a large tumor, parts of which may be firmly adherent to
the facial nerve. If the facial nerve is being heated by electrocoagulation, or heated by the drilling of bone adjacent
to the facial nerve, transient or sustained facial muscle
activity will result. Although such monitoring of facial
EMG activity is important in reducing the risk of permanent
damage to the facial nerve, it must be pointed out that the
facial nerve can be injured permanently from surgical
manipulation without any EMG response being noted; thus, injury
from sharp dissection will most likely not result in recordable
EMG activity (or any movement of the face). For this reason,
when the surgeon is dissecting near the facial nerve spontaneous
EMG activity should not be relied upon for assurance that the
facial nerve remains intact; in this situation, electrical
stimulation of the facial nerve should be used frequently to
identify the facial nerve so that the surgeon remains aware at
all times of the exact location of the facial nerve.
 |
Monitoring of
the Extraocular Nerves during Skull Base Surgery |
 |
In
operations to remove tumors of the skull base, several cranial
motor nerves are often in the operative field and are thus at
risk of injury from surgical manipulation. This is particularly
true in operations within the cavernous sinus, where the nerves
that innervate the extraocular muscles [the oculomotor (CN
III), trochlear (CN IV), and abducens (CN VI) nerves] may be
difficult to identify visually or may be displaced by the tumor.
Recording EMG potentials from the extraocular muscles while the
surgical field is being probed with a hand-held stimulating
electrode similar to that used for stimulating the facial nerve
(Fig-1C) can aid the surgeon in locating the respective
nerves. EMG potentials can easily be recorded from these
muscles via needle electrodes inserted percutaneously into the
respective muscle (Fig-2). The potentials recorded from the extraocular muscles in response to electrical stimulation of the
respective nerves are easy to distinguish (Fig-3A).
More
recently, surface electrodes for recording EMG activity from the
extraocular muscles have been developed. Facial nerve function
should also be monitored in these operations, using the methods
just described. Continuous recording of EMG potentials from
these muscles is also important, because injury to the respective nerves from mechanical manipulation and from heat
during electrocoagulation will often result in transient or
sustained EMG activity, as was described for the facial nerve.
Thus, such activity can be an important aid in preserving these
nerves. |
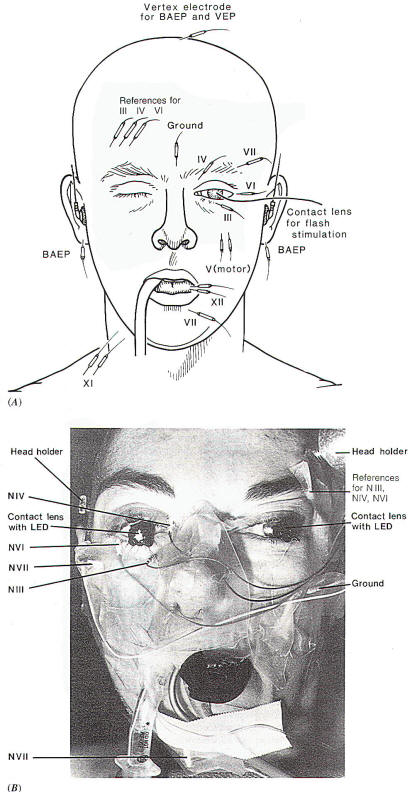 |
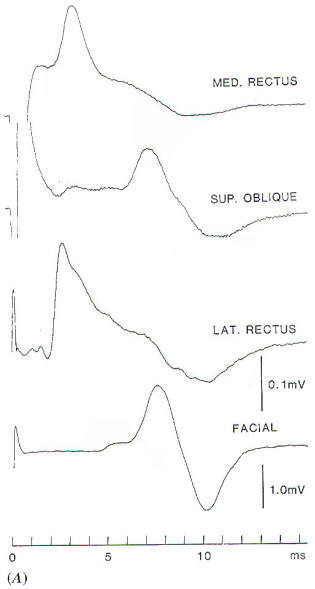 |
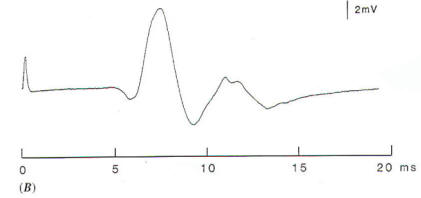 |
Fig-2: A-Electrode
placement for recording from facial,
extraocular muscles and the tongue. The
reference electrodes are all placed on the
forehead on the opposite side, to avoid
recording from the facial muscles at the
same time. B-Electrode placement with
intraoperative recordings from extraocular
and facial muscles. BAEPs and VEPs included. |
Fig-3: A- EMG potentials
recorded from the extraocular and facial
muscles in response to intracranial
electrical stimulation of the respective
cranial nerves, using the electrode
arrangement as seen in Fig-2. B-EMG
potentials from the tongue in response to
stimulation of the hypoglossal nerve. |
 |
Monitoring of
Other Cranial Motor Nerves |
 |
Identification of the hypoglossal nerve (CN XII) can be
facilitated by recording EMG potentials from the tongue (Figs-2A and
3B), and monitoring of the accessory nerve (CN XI) can
conveniently be done by placing pairs of needle EMG electrodes
in the trapezius muscle (Fig-2A). The motor portions of
the glossopharyngeal (CN IX) and vagus (CN
X) nerves can be monitored by
stimulating the respective nerves electrically and recording EMG
activity from the muscles that these nerves innervate in a way
similar to that just described for the facial nerve and the
nerves that innervate the extraocular muscles. A pair of
needle electrodes placed in the soft palate will record the EMG
response to stimulation of the glossopharyngeal nerve and
electrodes placed in the supraglottic region are suitable for
recording of the EMG response from laryngeal muscles that are
innervated by the recurrent nerve of the vagus nerve. Electrodes
placed on the endotracheal tube can record surface EMG
potentials from laryngeal muscles. A balloon placed on the tip of an endotracheal tube
as a pressure recording device has been used to record
contractions of the laryngeal muscles for monitoring of the
recurrent laryngeal nerve. This technique could be useful in
monitoring the more central portions of the vagus nerve.
One should
be aware when monitoring the glossopharyngeal, vagus, and
accessory nerves using electrical stimulation that there are
risks involved. For example, a supramaxial stimulation of the
accessory nerve may result in so strong a muscle contraction
that dislocation of joints or physical injury to muscles and
tendons may result. Electrical stimulation of the
glossopharyngeal and vagus nerves may cause cardiovascular
effects and should therefore be done cautiously.
 |
Monitoring of
Facial Nerve Function during Microvascular
Decompression for Hemifacial Spasm |
 |
Microvascular decompression to relieve hemifacial spasm is one
of only a few operations in which intraoperative
neurophysiologic monitoring can aid the surgeon in achieving the
therapeutic goal of the operation. It is generally
accepted that hemifacial spasm is caused by vascular compression
of the facial nerve as it exits the brain stem. and that
microvascular decompression of the root exit zone of the facial
nerve is the most effective treatment of this
disorder. However, it is not always obvious from
an exploration of the root exit zone of the facial nerve which
of several vessels is causing the spasm, and a certain (small)
number of patients who have undergone this operation have
experienced spasm postoperatively. Some of these patients had to
be reoperated upon, depending on the severity of the spasm.
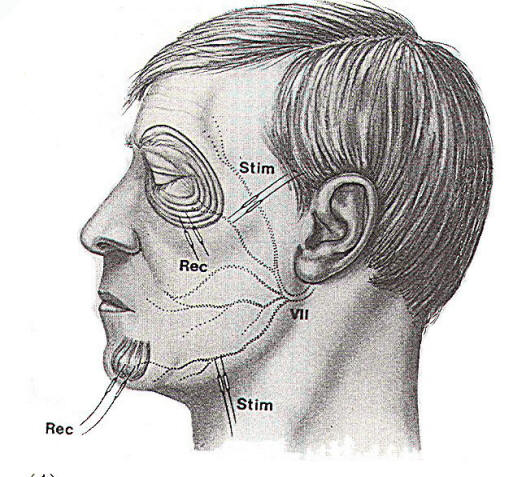 |
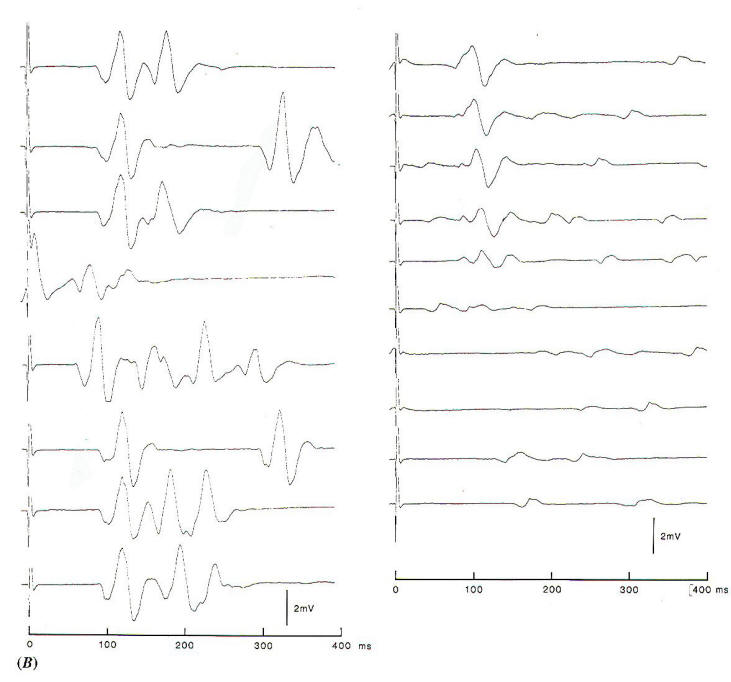 |
Fig-4A: Electrode placement for
recording the abnormal muscle response in hemifacial
spasm. |
Fig-4B: The abnormal muscle
response recorded from the mentalis muscle to
electrical stimulation of the temporalis branch. The
left record is before opening the dura, showing
variable EMG activity in addition to component with
a latency of 10 ms. After decompression, the low
amplitude spontaneous activity is indicative for
slight facial nerve injury. |
In studies of the pathophysiology of hemifacial spasm, it was found that
an abnormal muscle response that seems to be characteristic of
the disorder disappears instantaneously when the offending
blood vessel is moved off the intracranial portion of the facial
nerve (Fig-4). This abnormal muscle response, which has
a latency of about 10 ms is seen when one branch of the
facial nerve is stimulated electrically and recordings are made
from muscles that are innervated by other branches of the facial nerve. By monitoring this abnormal muscle response intraoperatively, it is possible to identify the offending blood
vessel and to ensure that the nerve has been fully decompressed
by watching for the cessation of the abnormal response (Fig.4B) When using this method
it was found that even veins can cause hemifacial spasm and
that in many cases there was more than one blood vessel
compressing the facial nerve. There are reasons to assume that
at least some of the patients who experienced only partial
relief from their symptoms before this type of monitoring was
introduced did so because more than one vessel was affecting the
facial nerve and only one of the offending vessels was moved off
the facial nerve during the operation.
 |
Monitoring of
Brain Stem Auditory Evoked Potentials |
 |
Intraoperative monitoring of BAEPs is commonly performed to
reduce the risk of hearing loss as a result of intraoperative
manipulation of the vestibulocochlear nerve in operations in
the cerebellopontine angle. This
is important in operations on acoustic tumors, in which hearing
preservation is anticipated, as well as in microvascular
decompression operations on cranial nerves and in other
operations in the cerebellopontine angle.
BAEPs are
commonly recorded between electrodes placed on the vertex and on
the earlobe (or mastoid) of the ear to which the sound is
applied. BAEPs are best elicited by click sounds presented at a
rate of 20 to 40 pulses/s (pps) at intensities of 100 to 110
peak equivalent sound pressure (Pe SPL), The normal BAEPs are
characterized by 5 to 7 vertex-positive peaks that are generated
as the different structures of the ascending auditory pathway
are successively activated (Fig.-5).
Because the
BAEPs are generated by fiber tracts and nuclei of the ascending
auditory pathway in the brain stem, the vestibulocochlear nerve,
the cochlear nuclei, and the lateral lemniscus being the most
important generators of BAEPS-recording of these
potentials is not only useful for detecting injury to the
vestibulocochlear nerve but may also be of value in operations
in which the brain stem is being manipulated or when circulation
to the brain stem may be compromised. The nuclei of
the ascending auditory pathway are sensitive to manipulations of
the brain stem, and there
are indications that BAEPs may be more
sensitive
in detecting such effects than
are changes in heart rate and blood pressure. A change in the latency of peak V while that
of peak III remains unchanged (increased III - V interpeak
latency) indicates an effect from surgical manipulation on
structures located in the region of the superior olivary complex
on either side or on the lateral lemniscus and its nucleus on
the side contralateral to the side on which the BAEPs are being
elicited (tumor side) (Fig-6). It is therefore more valuable
to monitor BAEPs during other operations in which manipulation
of the brain stem may occur, such as the removal of large
acoustic tumors or other tumors of the cerebellopontine angle.
|
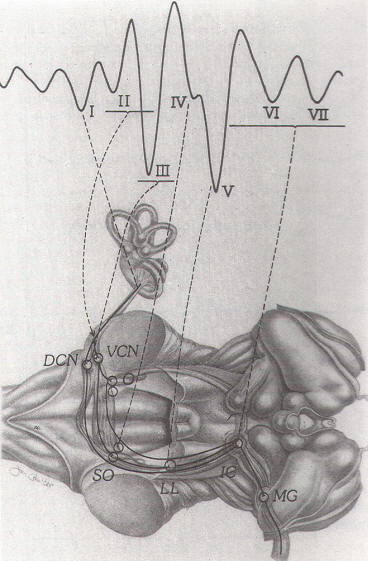 |
Fig-5: Neural generators
of BAEPs. DCN: dorsal cochlear nucleus, VCN:
ventral cochlear nucleus. SO: superior
olivary complex. LL: lateral lemniscus. IC:
inferior colliculus. MG: medial geniculate. |
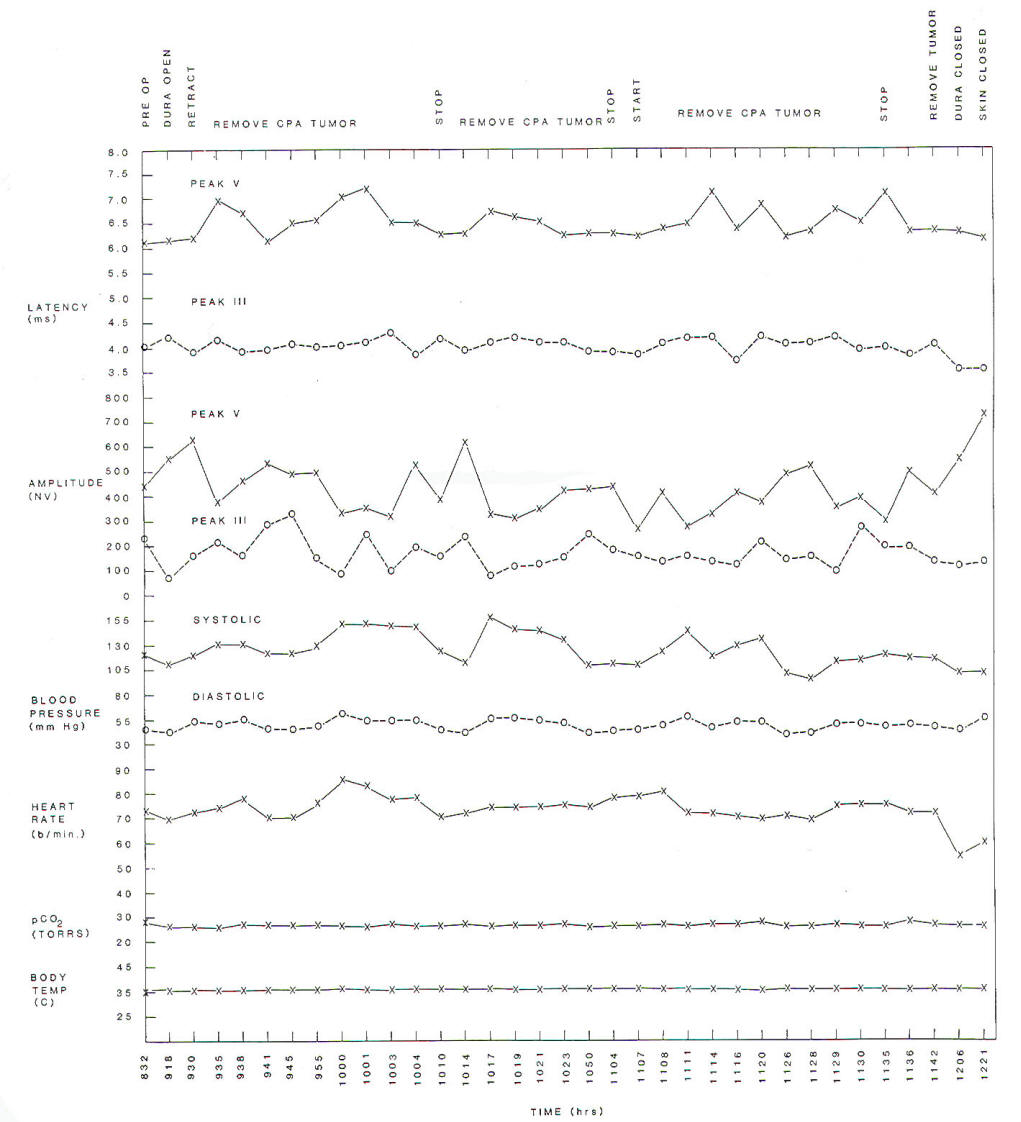 |
Fig-6:
Changes of latencies and amplitudes of peaks
III and V of BAEPs as a function of time
during surgery. BAEPs were elicited by
stimulating the opposite ear relative
to the tumor location. |
 |
Monitoring of
Somatosensory Evoked Potentials |
 |
SSEPs are
important in monitoring sensory conduction in the spinal cord. SSEPs elicited by stimulation of the median
nerve and recorded from the contralateral parietal region of the
scalp (C3 or C5) using a noncephalic reference are characterized
by a series of positive and negative peaks (Fig-7). P9, P11,
and PI4 are short-latency, positive peaks that are generated at
the level of the brachial plexus (P9). spinal entry (P11). and
termination of the dorsal column in the dorsal column nuclei
(PI4). The bilateral N18 is generated in brain stem
nuclei, such as the superior colliculus. whereas the
contralateral N20 is assumed to be generated in the primary
cortex. The waveform of the SSEPs and the presence of certain
components depend on the recording sites (Fig-7). For
monitoring the spinal cord, SSEPs are elicited by electrical
stimulation of sensory nerves on the leg (peroneal or posterior
tibial nerve).
Only when the cervical spine is
considered is it appropriate to use SSEPs elicited by
stimulation of the upper limb (median nerve at the wrist). The
response to stimulation of the lower limb is recorded from
electrodes placed on the scalp, vertex to a midline front
reference or vertex to a noncephalic reference. The
different components appear with longer latencies than those of
SSEPs elicited from the median nerve. and the pattern of the
peaks is more complex (Fig-8). One reason that lower limb SSEPs
are more complex than those elicited from
the upper limbs is related to the fact that
ascending neural activity elicited by
stimulating lower limbs travels in two
separate fiber tracts in the spinal cord.
Only part of the sensory information is
relayed in the dorsal column nuclei (nucleus
gracilis).
|
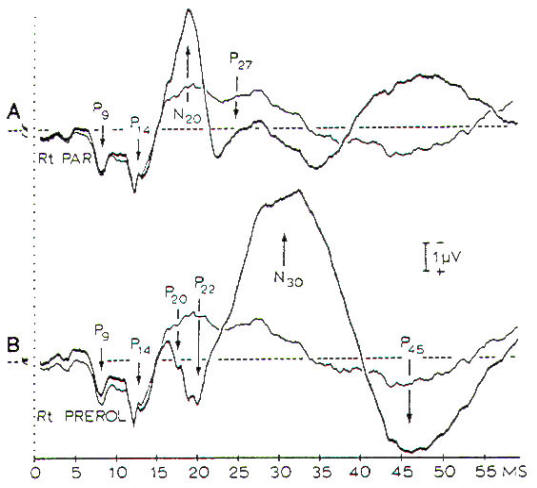 |
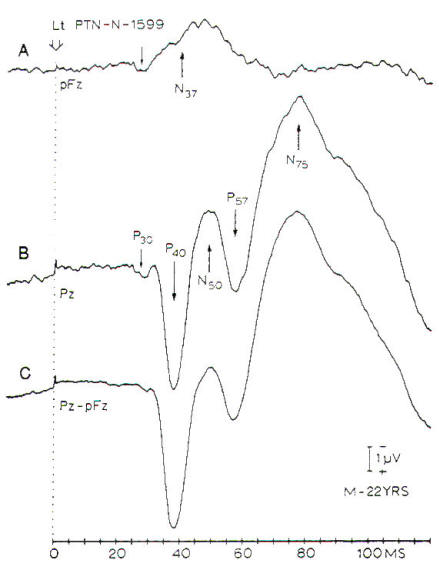 |
Fig-7:
SSEPs in response to electrical stimulation
of the median nerve. A- The thick line is a
record from the contralateral parietal
region with a noncephalic reference. B-
Records from prerolandic region with a
noncephalic reference.
|
Fig-8:
SSEPs in response to stimulation of the left
posterior tibial nerve at the ankle.
A-Records from a midfrontal (pFz).
B- Record from midpaietal (Pz)
with a noncephalic reference on the
shoulder. C- Difference between A and B.
|
Neural activity that travels in the dorsal
column is mainly elicited by skin receptors. Fast-conducting
afferents that conduct activity elicited by stretch receptors,
group Ia and II afferents from muscle spindles, and tendon
organs travel in a spinothalamic pathway in the dorsal lateral
funiculus of the spinal cord and synapse in the nucleus
gracilis, after which they join other afferents in the medial
lemniscus traveling toward the thalamic relay nuclei. The slow
cutaneous afferents from the lower limbs that travel in the
dorsal column are relayed in the dorsal column nuclei (gracilis
nucleus). These afferents have a large range of conduction
velocities, which causes a temporal dispersion in the elicited
activity, which is the cause of the low amplitude of the early
response from stimulation of lower limbs. This makes the SSEPs
from lower limbs qualitatively different from the SSEPs elicited
by stimulation of upper limbs and, together with the longer
distance from the location of the stimulation to the brain stem
structures, explains why the far field SSEP responses to
electrical stimulation of lower limbs are less well defined than
the SSEPs elicited by stimulation of the upper limbs, where all
somatic afferents trawl in the dorsal column and are all relayed
in the dorsal column nuclei (cuneate nucleus). Although
recording using a noncephalic reference is appropriate for
identifying the neural generators of SSEPs, the unfavorable
signal-to-noise ratio in such recordings has made it more common
to place the reference electrode on the scalp when SSEPs are
used for intraoperative monitoring. This reduces the pick-up of
electrical interference signals. When the spinal cord is to be
monitored, all components of SSEPs that originate from
structures that are rostral to the location where injury may
occur can be utilized for detecting injuries.
However, late components of
the SSEPs are affected by anesthesia, and it would
therefore be advantageous to use early components
such as the P14 in upper limb SSEPs.
Unfortunately, the early
components of lower limb SSEPs are less well-defined and usually
cannot be used for intraoperative monitoring.
Because the
blood supply to the portion of the spinal cord that comprises
the ascending somatosensory pathway is different from that of
the descending motor tracts, there is a possibility that the
motor system can be injured without any noticeable change
occurring in SSEPs. Thus, it seems possible that severe injury
could go unnoticed when only SSEPs are monitored. Although it
has been disputed whether this is in fact a real risk, there is
a need to be able to monitor both motor and sensory systems
during operations in which the spinal cord is at risk. Although
traditional techniques can be used to monitor the somatosensory
system intraoperatively, there are considerable technical
obstacles in eliciting motor responses upon stimulation of the
motor cortex. Therefore, such monitoring of the descending motor
tracts is not done routinely. It has, however, been shown that
it is indeed possible to elicit motor responses by electrical
and magnetic transcranial stimulation of the cortex,
thus a prerequisite for developing routine methods for
intraoperative monitoring of motor tracts.
Intraoperative monitoring of SSEPs is valuable as an indicator
of decreased cerebral perfusion in regions of the brain on which
the generation of more long-latency components of the SSEPs
depend. The development of these methods, which are now in
routine use, had been pioneered by Symon et. al, who made use of recordings of SSEPs elicited by electrical
stimulation of the median nerve at the wrist. The use of this
method is based on the finding that there is a rather close
relationship between the disappearance of electrical activity
and a decrease in the cerebral blood flow to 15 to 18 ml/100 g
per min (or below), and there are changes in the late components
of the SSEPs that occur before the perfusion reaches these low
levels. SSEPs are therefore valuable in estimating changes in
cerebral blood flow.
The most
useful parameter of SSEPs as an indicator of a decrease in
cerebral blood flow is the central conduction time, which is
the time difference between the occurrence of the components of
the SSEPs that can be recorded at the neck (P14) and the N20
component that is recorded at
the contralateral parietal skull (C3-C5) (Fig-9). Central conduction time is not affected
by changes in peripheral neural conduction or by
age, and it has been shown
to correlate with cerebral blood flow when blood flow is reduced
below a certain value.
|
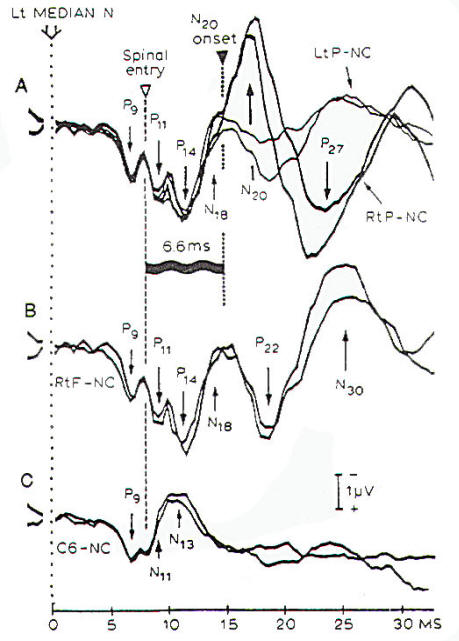 |
Fig-9: Measures of
central conduction time from recordings of the SSEPs
that are elicited by electrical stimulation of the
median nerve at the wrist. All recordings were done
with a noncephalic reference. A- Parietal scalp. B-
Frontal scalp. C- Spinal C6 spine. |
 |
Monitoring of
Visual Evoked Potentials |
 |
During
operations in which the optic nerve or the optic tract is being
manipulated it would seem to be beneficial to monitor
VEPS. However, the results of such monitoring have been
generally disappointing because the changes in the recorded
potentials correlate poorly with postoperative changes in
vision. This is most likely due to limitations inherent in the
techniques currently available for intraoperative stimulation of
the visual system, and perhaps to inadequate knowledge of how to
interpret the changes in the VEPs that may occur during surgical
manipulations of the optic nerve or optic tract: however,
essentially there are no practical problems involved in
recording VEPs intraoperatively. At present, the only
practical type of visual stimulation that can be used intraoperatively is the "flash" type. It has been shown that
VEPs elicited by a changing pattern (pattern
reversal-checkerboard pattern) are much more useful
diagnostically than VEPs recorded in response to flash
stimulation, however, eliciting VEPs by pattern reversal
technique requires that a pattern be focused on the retina,
which is not possible to accomplish intraoperatively.
 |
Effects of
Anaesthesia |
 |
Although
recordings of BAEPs are not noticeably affected by any common
anesthetic regimen using inhalation anesthetics. barbiturates.
and other intravenous anesthetics, intraoperative
monitoring of cranial motor nerves cannot be done if the
patient is paralyzed, because such monitoring depends on
recording muscle responses by EMG technique. Components of the
upper limb SSEPs that occur with latencies longer than 16 ms are
usually affected by general surgical anesthesia.
The most
commonly used anesthetic regimen for neurosurgical operations
involves giving a strong narcotic, such as fentanyl for
analgesia together with an inhalation agent, such as nitrous
oxide, and small amounts of halogenated agents ("balanced
anesthesia"). Because the patient must be kept from moving,
he or she must be paralyzed when such a regimen is used. Normally
some form of a muscle endplate blocker
(pancuronium, vecuronium.
etc.) is used for this purpose. Therefore, balanced anesthesia
makes it impossible to record EMG potentials because of muscle
relaxation. However, intraoperative neurophysiologic monitoring
is fast becoming a regular component of the surgical arena, and
it is now common to adjust the anesthesia regimen to the
requirements of the specific type of monitoring to be done.
For instance, the requirement of being able to record EMG
potentials has resulted in the use of inhalation anesthesia
throughout the operation. possibly with the addition of a small
amount of a narcotic agent and benzodiazepines such as midazolam.
This type of anesthesia has been used for many
years without any noticeable difficulties or complications and
is now in common use when intraoperative monitoring of muscle
activity is performed. However, while such an anesthesia regimen
has no noticeable effect on recording EMG potentials or BAEPs
and short-latency SSEPs. it is likely to suppress later
components of SSEPs and it greatly suppresses motor evoked
potentials. Some anesthetic agents such a propofol,
that can be
administered intravenously have less suppressive effect on
cortical responses and are thus being used during operations in
which several different kinds of evoked potentials and EMG
responses are to be monitored.
 |
Preoperative
Assessment of Patients for Intraoperative
Monitoring |
 |
Patients in
whom intraoperative neurophysiologic monitoring of evoked
potentials is to be performed should have the systems that are
to be monitored quantitatively evaluated preoperatively. For
example, if a patient's BAEPs are to be monitored, his or her
hearing status should be evaluated preoperatively. Testing
should include at least a pure tone audiogram and a
determination of speech discrimination scores, and the patient's
ear canals should be checked for obstruction (from cerumen. etc.).
If the patient has no
hearing or if it is not possible to obtain an interpretable BAEP
before the operation, it will not be possible to obtain one
during the operation. If, on the other hand, an interpretable BAEP can be obtained before the operation but not
intraoperatively. the cause is most likely technical: such
problems then must be corrected. Similarly, if SSEPs are to be
recorded intraoperatively, a recording should be performed
preoperatively to ascertain that the patient has normal SSEPs or
to establish the degree of abnormality so that it can be taken
into consideration before the intraoperative recordings are
made. Factors such as neuropathies that may affect neural
conduction should be ruled out or assessed quantitatively, if
possible. If the extraocular museles are to be monitored, their
function should be evaluated preoperatively by
state-of-the-art methods. When facial EMG responses are to be
monitored, the function of the facial musculature should be
evaluated preoperatively. Preoperative evaluation of sensory and motor systems that may be at risk during an operation is also
essential, because this, in connection with similar tests done
postoperatively, the only way that deficits from the
operation can be assessed quantitatively.
 |
Determination
of Benefits of Intraoperative Neurophysiologic
Monitoring |
 |
Intraoperative neurophysiologic monitoring of evoked potentials
has been introduced for use in neurosurgical
operations for long time, and it is therefore natural that the method has
been viewed with skepticism in the early period of its
implementation, and suffered from a demand for proof of the
benefits. As with several other procedures allied
to such operations, it has generally been difficult to design
studies to provide quantitative estimates of the benefits in
terms of reduced postoperative complications. This is largely
because many surgeons believe that intraoperative monitoring is
valuable in reducing postoperative deficits and therefore will
not allow random selection of patients to be monitored. This
makes it impossible to study the efficacy of such techniques by
a double-blind methodology.
Comparing
complications before and after the introduction of
intraoperative monitoring thus seems to be the only practical
way to assess the value of intraoperative monitoring: however,
the results of such evaluations are influenced by any changes
in the operative technique introduced at or after the
institution of monitoring. Despite this complication.
comparisons have been made between the rate of complications
(such as facial palsy) of surgical procedures (such as acoustic
tumor removal) before and after the introduction of intracranial
monitoring of cranial nerve function. Such studies have shown a
significant decrease in complication rate after the introduction
of intraoperative monitoring.
It is
generally accepted now that intraoperative monitoring of facial
nerve function during operations on acoustic tumors is of value
in preserving the facial nerve. A study of a less frequently
performed operation, that to relieve hemifacial spasm, showed
that the efficacy of the operation was higher after
intraoperative monitoring was introduced. Further, the number
of patients who needed to be reoperated upon because of
recurrent or unrelieved spasm decreased from about 15 percent to
almost zero. Finally, the frequency of hearing loss as a
complication of microvascular decompression operations in the cerebellopontine angle decreased radically after the
introduction of intraoperative monitoring of BAEPs during such
operations.
Because
intraoperative neurophysiologic monitoring can many times
identify exactly which step in an operation that caused an
injury is likely to result in a permanent neurologic deficit, it
has contributed to the development of better and safer operating
techniques. When evaluating the benefits of intraoperative
neurophysiologic monitoring it must be pointed out that the
benefit from intraoperative neurophysiologic monitoring depends
on the level of experience of the surgeon. Thus a very
experienced surgeon will not have the same degree of benefit
from this technique as might a less experienced surgeon. There are several advantages of the use of
intraoperative neurophysiologic monitoring, but they are
difficult to measure quantitatively; nevertheless, they are
great enough to lead most surgeons who have operated with the
aid of such monitoring to demand that it continue.
|
 |
|